Aquifer storage and recovery
Excess fresh water is infiltrated and stored in aquifers through wells or infiltration ponds, and recovered from the same wells when needed. Aquifer storage is increasingly applied worldwide.
Thema |
Zoetwatervoorziening, Delta facts, English versions |
Tags |
|
Downloads |
- INTRODUCTION
- RELATED TOPICS AND DELTA FACTS
- STRATEGY: HOLD, STORE, USE!
- SCHEMATIC
- TECHNCAL SPECIFIATIONS
- GOVERNANCE
- COSTS AND BENEFITS
- FIELD EXPERIENCE AND CURRENTLY ACTIVE RESEARCH
- KNOWLEDGE GAPS
- EXPERIENCES
Introduction
Aquifer storage and recovery (ASR) is defined as "the storage of water in a suitable aquifer through a well during times when water is available, and recovery of the water from the same well during times when it is needed" (Pyne, 2005). Essentially, excess fresh water is infiltrated and stored in aquifers through wells or infiltration ponds, and recovered from the same wells when needed. Increasingly applied worldwide, it is often considered the technology of choice because (Zuurbier et al., 2012a):
- Water can be stored for extended periods of time and recovered as needed;
- There is insufficient aboveground storage space;
- Water is well preserved, e.g. no algae growth;
- The stored water is protected from external influences, such as temperature fluctuations, evaporation, contamination;
- It eliminates the need for (expensive) water treatment and prevents the build-up of waste streams;
- It helps create aboveground space for collection of peak precipitation and prevents the loss of precious fresh water.
The technology is used in areas where the original groundwater quality is poor due to high salinity levels, or where the quality is suitable, but net extraction can cause damage as a result of dehydration. In the Netherlands, this technology is applied primarily at drinking water companies (Stuyfzand, 2005), and increasingly in the greenhouse sector (Zuid-Holland, 2009), where large quantities of rainwater are captured on the greenhouse roof and used as an ideal and low-cost source of tap water for irrigation.
Related topics and Delta Facts
Keywords: fresh water supply, salinity
Delta facts: Rainwater lenses, Soil as a buffer, Company water reservoirs, Brackish seepage.
Strategy: hold, store, use!
The primary purpose of aquifer storage and recovery is to bridge periods of supply and demand imbalance. It helps increase fresh water availability during periods of drought, thereby preventing damage in agriculture and horticulture through the use of fresh water for irrigation. In short, aquifer storage and recovery allows for effective use of the annual fresh water surplus.
It contributes to water safety and helps prevent flood events resulting from heavy precipitation in urban areas by creating storage space through level management in aboveground basins. Precipitation can therefore be captured more effectively aboveground and slowly infiltrated, ensuring availability of a source of fresh water in dry periods.
Schematic
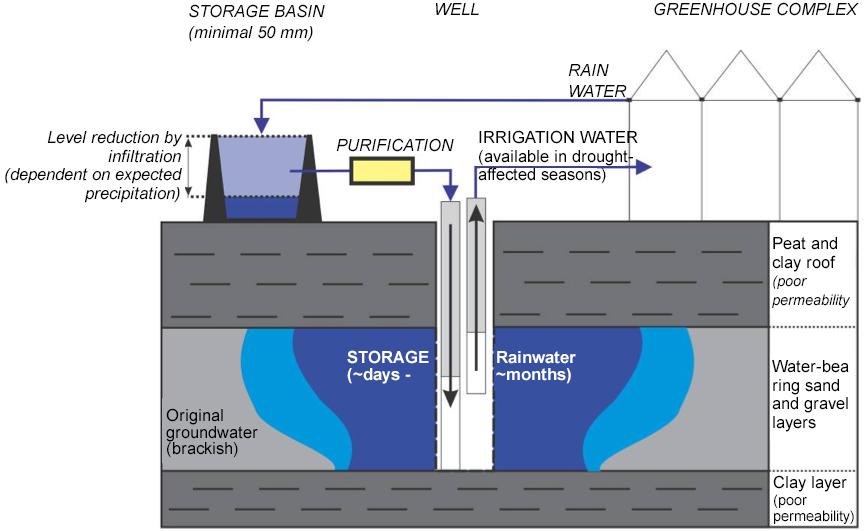
Figure 1. Schematic of aquifer storage and recovery (ASR) for irrigation needs of greenhouses.
Technical specifications
Intercepted rainwater, collected surface water and/or purified wastewater is injected in aquifers when there is a water surplus. Rainwater and/or surface water is additionally purified (for example, through a sand filter) to remove suspended solids and prevent well clogging. When fresh water supply and demand are in balance, water is neither injected nor extracted, making it a 'storage phase'. When water shortage occurs, the fresh water is extracted from the wells for use as drinking or irrigation water. This process can be fully automated. In the greenhouse sector, for example, this is done by means of monitoring the water level in the rainwater storage system and if the maximum level is exceeded, infiltration commences. If the minimum level is breached (because of irrigation in the greenhouse) the system will start tapping water.
In aquifer storage and recovery, the recovery rate (the percentage of injected water of acceptable quality that can be recovered) is obviously important. The recovery rate can be disappointing, however, particularly in brackish or saline aquifers, because:
- The injected fresh water flows horizontally in an area with considerable background flow, and highly saline (unsuitable) water is recovered from the upstream side;
- The injected fresh water floats to the top of the saline water, and saline water is recovered from the bottom of the well(s), making the mixed water unsuitable for recovery.
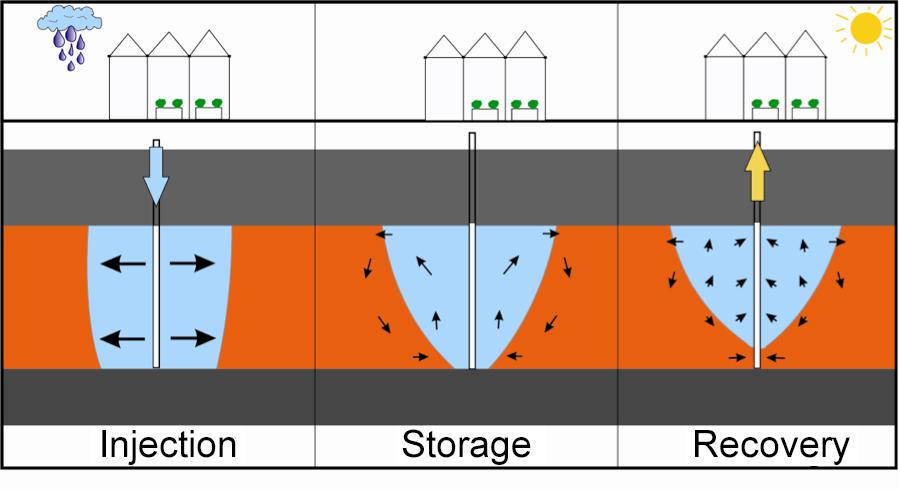
Figure 2. Injection, storage and recovery of fresh water in a brackish or saline aquifer, where salinity at the bottom of the well ultimately renders the recovered water unsuitable.
Because of the aspects mentioned above (floating/displacement), the recovery rate depends on the location. Important control parameters are the aquifer’s thickness and permeability ('coarseness'), salinity of the original groundwater, background flow rate and closure of the target aquifer as a result of the layers of clay and peat at the top and bottom of the aquifer.
The scale is also important: injection of a large quantity of water will cause a large bubble to form and thus increase the recovery rate. In other words, the size of the fresh water bubble reduces the chances of successful aquifer storage and recovery in areas with brackish or saline groundwater. The minimum scale for successful aquifer storage and recovery is therefore a good indicator of the extent to which this technology is suitable for application in particular regions. In Greenport Westland-Oostland, for example, small-scale systems prove especially suitable in the inland region of Oostland, while upscaling is required in the coastal region of the Westland (Zuurbier et al., in press).

- Figure 3. Required injection flow for successful aquifer storage and recovery in Greenport Westland-Oostland (Zuurbier et al., in press).
In addition to upscaling, recent research shows that the well configuration can also increase the recovery rate. Use of multiple partially penetrating wells in one borehole proves advantageous, as it allows the preferential fresh water to be injected to the bottom of the aquifer, while limiting recovery to the top of the aquifer. This slows down salinity build-up in the recovery wells and significantly increases the recovery rate (Zuurbier and Stuyfzand, 2012b; Zuurbier et al., submitted).
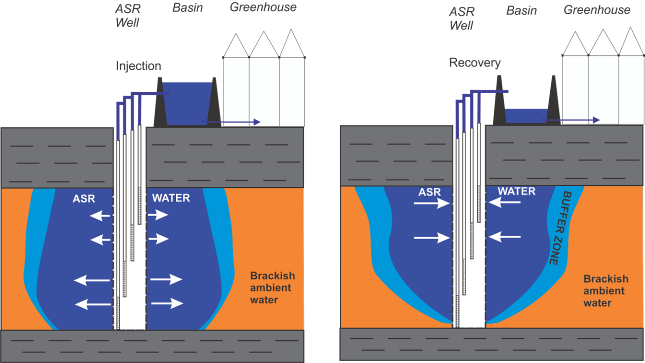
Figure 4. Use of multiple partially penetrating wells in one borehole for injection and recovery of fresh water in brackish aquifers (Zuurbier and Stuyfzand, 2012b).
Further optimisations are possible, for example, by extracting the upconed brackish water to prevent it from reaching the extraction wells (interception well or 'fresh-keeper concept'). Because it is unsuitable for use, the water must be discharged (if possible), desalinated or re-injected elsewhere in the groundwater (e.g. Van der Valk, 2011; Van Ginkel, 2007; Vink et al., 2010).
Governance
In the current groundwater management policy, the Water Boards are the competent authority for aquifer storage and recovery for irrigation purposes, while the Provincial Councils are the competent authority for drinking water applications.
Based on the advice of the Technical Committee on Soil Protection (Technische Commissie Bodem, 2009) and the policy of the province of Zuid-Holland and the Delfland Water Board, the following can be concluded about underground infiltration and extraction of rainwater (Zuurbier et al., 2012a):
- The aquifer infiltration and extraction technology can also be applied under certain policy conditions, hence the permits that have been issued for various locations in the Netherlands (e.g. locations in the greenhouse region of Oostland, Agriport A7, etc.);
- The Technical Committee on Soil Protection is reticent in its advice regarding rainwater (meteoric) infiltration. An important consideration is the concern that pollutants may migrate from the rainwater to deeper groundwater supplies. This is in line with the policy pursued at the Delfland Water Board, which states that there should be no potential for adverse effects to occur. To ensure sustainability of an infiltration system, the injected water must be as clean as possible. Thus, fine-grained sediment particles, which trap most pollutants, must be removed before injection takes place. This also prevents well clogging.
- The area-specific calculations to be performed during the permit application process will clearly show whether adverse effects are expected with respect to the use functions and/or other systems. The Delfland Water Board has prepared a checklist for this purpose. In practice, few adverse effects have occurred in the systems that are already licensed.
To date, use of the subsoil aquifer has been controlled and regulated to a very limited extent, and aquifer storage and recovery competes with other uses of aquifers, such as thermal energy storage (TES, also commonly called heat and cold storage) and extractions and injections for reverse osmosis plants. Given the benefits, but also the specific requirements for aquifer storage and recovery systems (which is less applicable to the other applications), it is recommended to use suitable preferential aquifers for aquifer storage and recovery in areas with high fresh water demand (such as greenhouse regions).
In addition, the injection water must be of such quality that sustainable use of the subsoil aquifer is guaranteed. This can be achieved through proper preparation of the injection water, for example, by separating the different water streams in the greenhouse (until only pure rainwater remains for injection) and selective intake of fresh surface water (outside growing/irrigation season). Further purification can be performed, if necessary. The question in that case, however, is whether the cost price will be competitive with that of other fresh water production methods. Monitoring is necessary for maintaining the quality of the injection water.
Costs and benefits
The costs of aquifer storage and recovery were recently calculated in two studies comparing the per-m3 cost price and benefits of storage of rainwater in greenhouses (Zuurbier et al., 2012a) using a simple system at a suitable location (low cost price, proven technologies) and a clustered system with an optimised well design at a less suitable location with a lower yield (higher cost price, technology in development). The studies found that:
- The cost price of aquifer-stored water is between € 0.20 and 0.70/m³ and is lower than drinking water (~ € 1.20/m³) and water produced through local desalination (reverse osmosis, € 0.60 to 1.00/m³);
- The quality is better than drinking, surface and basin water;
- There are no charges for disposal of waste streams.
A cost price of € 0.35/m3 is calculated for aquifer storage of fresh surface water in Zeeland, using the so-called 'Freshmaker’ technology (Vink et al., 2010). This is more cost-effective than water supplied from the local agricultural water line of Evides (approximately € 0.60/m³). This technology is still in its infancy, however (see 'Field Experience and Overview Currently Active Research’).
Field experiences and currently active research
In areas where the technology is known to be viable and water is well preserved in the aquifer, application has been taking place for some time. Examples of these areas are Oostland (in the vicinity of Bleiswijk, since the 80s), Aalsmeer and Wieringermeer (since ~ 2007). In addition, in areas where aquifer storage and recovery has less potential (Zeeland, Westland), alternative methods are being explored to identify the best methods possible to recover fresh, stored water. Key pilots (all performed within Knowledge for Climate) are highlighted below:
Optimal, small-scale aquifer storage and recovery in brackish aquifer: Nootdorp field trial
In an orchid nursery (2 hectares, water demand ~50% of precipitation) in Nootdorp, a study was conducted into whether self-sufficiency can be achieved in supplying fresh irrigation water by storing the fresh water surplus in winter in a brackish aquifer. Recovery of the stored fresh (meteoric) water is theoretically limited here due to the spontaneous upconing of the small fresh water bubble in the brackish aquifer (Figure 2). A well system that, in theory, should significantly improve the recovery rate of the aquifer was tested in practice with grower and installer B-E De Lier.
The new type of aquifer storage and recovery system was installed between November 2011 and January 2012. The site is located in the northwest of the Oostland region at about -4 m-NAP; an area with brackish and relatively stagnant groundwater in the chosen aquifer (Figure 5). The four-well system is equipped with control valves flow meters and EC meters. Three casing nests are installed to monitor the injection water in the aquifer. A 27m-long core of sediment was taken from the target aquifer, for description by TNO, and for analysis of grain size, carbonate and organic particle concentration and elementary composition (XRF).
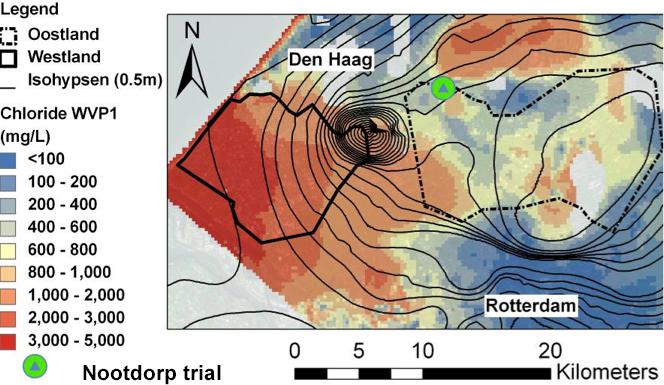
Figure 5. Location of the Nootdorp field trial. Chloride concentrations in the target aquifer are based on Oude-Essink et al. (2010).
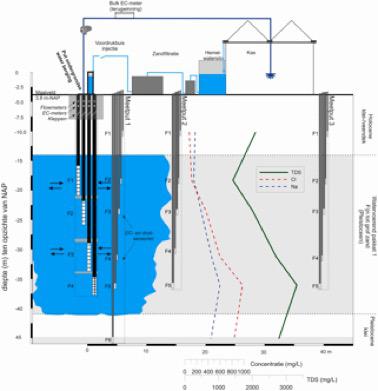
Figure 6: Cross section of the field trial shows the logistics of the aquifer storage of the recovery system and the measurement points, as well as the chemical makeup of the original groundwater. Cl = chloride, TDS = total dissolved solids.
INJECT FROM THE BOTTOM, RECOVER FROM THE TOP
Injection of fresh water starts automatically when precipitation in the rainwater silo exceeds a predefined level, while recovery takes place when the irrigation silo (daily supply) falls below its defined minimum level. The depth at which water is injected and recovered is flexible due to the underground four-well system and aboveground control. After an initial injection and recovery phase through all filters, a transition is made to a schedule where fresh water is injected deep into the aquifer and recovered from the top so as to keep the brackish water moving upwards by pumping and seepage as far away from the recovery wells for as long as possible.
In the first year, this led to recovery of very fresh water (chloride <18 mg/l) that exceeded 40% of the injected water which, based on the modelling, would have been no more than 15% had a conventional well been used. In the coming years, this efficiency will increase to about 60% (figure 7) which is more than enough to provide the grower with sufficient fresh water. A higher efficiency level is not achievable with this small-scale system: about 40% of the water will structurally be lost when the water sources are mixed as the brackish water is pushed back to the bottom. Installation of a short single well filter in the upper half of the aquifer would have increased the yield. However, in that case, the yield would have remained at ~35%, a significantly lower yield than the current configuration in operation.
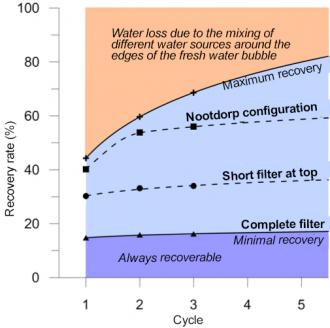
- Figure 7. The recovery rate of the different well configurations as modelled for the Nootdorp field trial. A small fraction (<20%) is easy to recover. The selected configuration, however, recovers ~60% of the injected water (Zuurbier et al., submitted).
The Nootdorp field trial will run for two years, after which the system will remain in operation for supplying irrigation water to the grower.
Optimal, large-scale aquifer storage and recovery in saline aquifer: Westland field trial
This field trial is similar to the Nootdorp field trial, with some major differences:
- The target aquifer is considerably more saline (over 4,000 rather than up to 1,000 mg/l);
- Four companies share the aquifer, and almost 30 hectares of greenhouse area are used for collecting rainwater ('upscaling');
- Preferential rainwater is injected through two boreholes (each with three filters) to the bottom and upstream into the aquifer, and recovered from the top and downstream;
- After salinization, the deepest filters can continue to be used for extracting (capturing of) the saline water build-up under the bubble in order to prevent salinity of the recovery wells ('fresh-keeper concept').
This field trial was launched in late 2012 and will run until (at least) 2014.
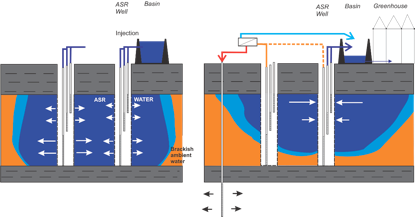
Figure 8. Purpose of the Westland field trial with two wells (each with three filters) and possible capture of deeper saline water. The saline water may optionally be desalinated to produce more irrigation water.
Optimal aquifer storage and recovery in a shallow saline aquifer with horizontal wells: The Freshmaker
This conceptual system was installed in Ovezande in the winter of 2012/2013 (Zuid-Beveland, Zeeland). Using two horizontal wells (constructed by horizontal directional drilling), the system:
- Infiltrates fresh water from a nearby fresh watercourse at 7m below the surface (during wet periods) after a brief period of purification;
- Captures saline water at ~15m below the surface and discharges it via surface water or injection into saline aquifer;
- Recovers fresh water for irrigation of an orchard (during dry periods).
Storage causes the natural 10m-thick fresh water lens to turn approximately ~5m thicker across approximately 70m. Fresh water will be injected for the first time in the spring of 2013, followed by recovery in the summer of 2013. Monitoring and modelling will be used to profile the efficiency of this fresh water storage system. Model calculations show that almost all the injected water can be recovered.
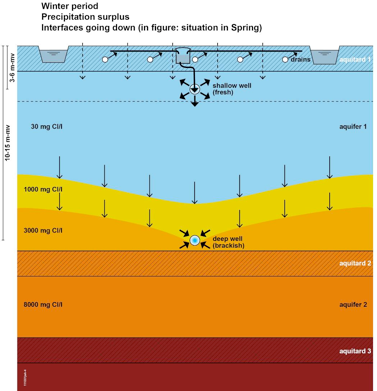
Figure 9. Freshmaker performance
Fresh water is injected at a shallow depth through a horizontal well, while saline water is extracted from greater depths from a second horizontal well. This enlarges the fresh water lens and fresh water is available in the summer.
Aquifer storage and recovery on high sandy soils: The Stippelberg
To test the feasibility of phreatic aquifer storage and recovery systems, a pilot study was conducted for the Stippelberg in 2012. The Stippelberg is a dry and dried out forest reserve to the east of the province of Noord Brabant. Aquifer storage and recovery holds promise for this area because (1) farmers, land managers and the water manager will gain added value from reduced drought sensitivity of the area, and (2) the sponge effect of this dried out natural reserve is expected to be utilised more efficiently, in part, due to isolating geological faults.
This study explores the potential of the following two concepts for utilising the sponge effect of natural reserves:
- Conserving the local precipitation surplus by shoaling regional drainage outlets
- Supplying and infiltrating external water by way of deep or shallow infiltration
Exploratory calculations will be performed to estimate the effectiveness of these measures. A field experiment on the effectiveness and impact of shallow infiltration is also set to start in May 2013. The objectives of this experiment are to verify (1) the effectiveness of this storage measure, and (2) the impact on groundwater levels and patterns in groundwater quality.
Knowledge gaps
- Upscaling: what are the expected recovery yields in different regions?
At present, the ASR technology has been widely proven in the vicinity of Oostland and Aalsmeer, with about 100 installed systems (the first systems date back to 1983). Studies on the efficiency of these systems are limited, however (Zuurbier et al., in press). In other areas where ASR is not yet in operation, but where the technology is being considered for counteracting water shortages, an estimate must be made of the expected recovery yields. The extent to which artificial infiltration of surface water can reduce damage caused by drought on the sandy soils (by irrigation and recovery of drinking water) is not quantified.
- Optimisation: what will upscaling and improved well configurations yield?
For the first time since 2011, a new approach has been taken within Knowledge for Climate to further develop and implement (in practice) theoretical improvements to ASR systems in brackish/saline areas. This approach provides quick insight into the capabilities of the system, as well as new inspiration for improvements. It is also clear that it takes time for new concepts to be (widely) applied in practice.
- Geochemical reactions and development of water quality
It is known that the quality of water changes during storage in the aquifer, rendering it unsuitable for drinking water. Metals and trace elements, such as arsenic, can remobilise from the sediment to aquatic systems. To what extent this renders the water unsuitable as irrigation water is still under investigation in the trials conducted within Knowledge for Climate.
- Legislation
Water managers (i.e. the Water Boards) know little about the performance and impact of ASR systems, and the regulations in force often fail to address the small-scale nature of the systems, resulting in potentially stringent monitoring requirements (that are set forth for large-scale infiltration in drinking water areas, for example) that render the storage system economically unattractive. Putting in place an appropriate regulatory framework that achieves the twin objectives of mitigating the risks of large-scale groundwater contamination, while making economically viable production possible, is therefore desirable.
Experiences
- Valuable experiences gained in greenhouse regions Oostland and Aalsmeer (relatively fresh water), where some 100 systems have been installed since 1983;
- Lower yields are forecast and experienced on a small scale in more saline areas, but could largely be counteracted by upscaling and improved well configurations;
- To date, the added value of such an improved well configuration has been quantified only in the Nootdorp trial within the Knowledge for Climate programme and is being further investigated for other locations/configurations;
- Change in the quality of water caused by reactivity of the subsoil can make the water unsuitable for drinking water. See, for example, the Herten ASR trial (WML drinking water company): water quality not suitable for drinking water due to mobilisation of metals (Antoniou et al., 2012). Solutions for preventing such mobilisation are being explored within TTIW/Wetsus (Sustainable subsurface storage of (drinking) water);
Good results achieved in Australia (since 2000) with respect to aquifer storage of treated effluent for use as irrigation water.